Conventional electronics is based on manipulating electrons through their charge. However, scientists are exploring other types of electron transport. Important results are now reported for orbitronics, in which the orbital angular momentum of electrons is exploited.
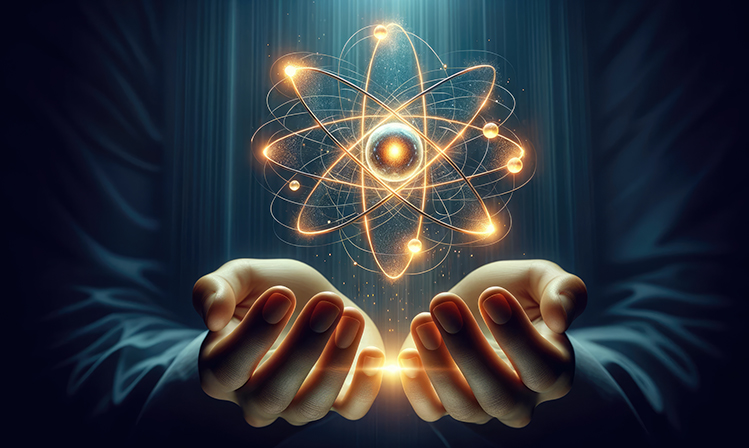
©︎ John― stock.adobe.com/jp
Conventional electronics is based on the manipulation of electrons in solids. Specifically, this technology uses the charge of electrons to control their flow in device components such as integrated circuits, transistors, and diodes. Besides charge, electrons have another intrinsic property called "spin." This spin is a form of angular momentum, or tendency for an electron to rotate around its own axis. "Spintronics" is a field of study developed on the notion that the spin of the electron is a quantity that can be used to control spin currents.
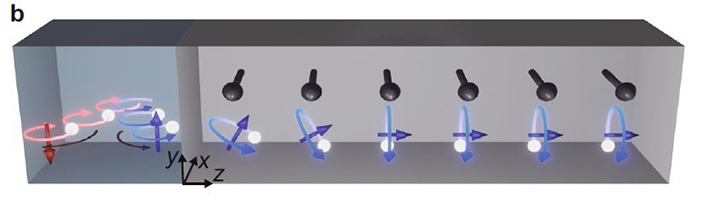
[Fig. 1b from the paper]
Orbital transport in a system consisting of a non-magnetic (left) and a magnetic (right) material.
(https://doi.org/10.1038/s42005-023-01139-7) is licensed under CC BY 4.0 DEED
Another level of electron manipulation is related to electron orbital angular momentum. This refers to a magnetic moment that arises when an electron describes an orbiting path such as that around an atomic nucleus. "Orbitronics," the associated type of electronics, is still a nascent technology and needs to be researched further before it can be implemented. Assistant Professor Hiroki Hayashi from Keio University and colleagues have published experiment results in which they were able to successfully achieve long-distance orbital currents as well as giant current-induced torques. These experiments used the orbital Hall effect which is a charge-orbital conversion phenomenon. These results signify an important milestone towards orbitronics-based technologies being used in the real world.
Assistant Professor Hayashi's experiments were conducted with nickel and titanium bilayers. The titanium layer acts as the source of the orbital currents. Working with titanium has the advantage of making it easier to distinguish the orbital effects from the effects from the electrons' spin. Titanium is also a more environmentally sustainable choice than other materials for producing orbital torque in nonmagnetic and magnetic bilayers, as it is light, abundant, and inexpensive.
When the orbital current is injected into the nickel layer, the electrons' orbital angular momentums interact with the intrinsic magnetic moments. Nickel is a ferromagnet like iron, meaning that it is attracted to magnets and can be magnetized. This interaction leads to torque being exerted on the nickel layer's magnetic moments, satisfying the law of conservation of angular momentum. Hayashi and his colleagues were able to demonstrate and measure this torque using a method called "spin-torque ferromagnetic resonance."
The researchers performed additional experiments with other nonmagnetic and magnetic bilayer combinations, such as Ni81Fe19 instead of nickel, and tungsten instead of titanium. They also varied the number of atomic layers when performing calculations so that they could create a comprehensive, quantitative overview of orbital responses in this scenario for future reference when fine-tuning their experiments.
The results unambiguously demonstrate long-range transport of angular momentum in nickel, especially with the nickel and tungsten bilayer which created a "giant" torque that is 10 times more efficient than other previously investigated materials in spintronics. These discoveries of large orbital torques in bilayer systems represent another important milestone towards practical orbitronics applications including magneto-electric spin orbit (MESO) devices which are a theoretical replacement for complementary metal-oxide-semiconductors (CMOS).
Quoting the scientists: "We...believe that our discovery of the long-range orbital transport and gigant orbital torque efficiencies provides important information for the material design of orbital-based devices, which will stimulate further experimental and theoretical studies and lead to the fundamental understanding of the physics of orbital currents for practical applications."-Keio research team
Published online 14 December 2023
About the researcher
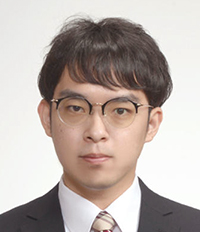
Hiroki Hayashi ― Assistant Professor (Non-tenured)
Graduate School of Fundamental Science and Technology
Hiroki Hayashi received a B.E., M.E., and Doctorate in
Applied Physics and Physico-Informatics from Keio University
in 2018, 2020, and 2023 respectively. He is currently an Assistant
Professor (Non-tenured) at the School of Fundamental Science
and Technology, Keio University.
His research interests are in spin and orbital dynamics in solids
as well as related phenomena in condensed matter physics.
Links
Reference
- Hayashi, H., Jo, D., Go, D. et al. Observation of long-range orbital transport and giant orbital torque. Commun Phys 6, 32 (2023). | article