Quantifying multi-exciton generation for solar energy conversion in molecular materials
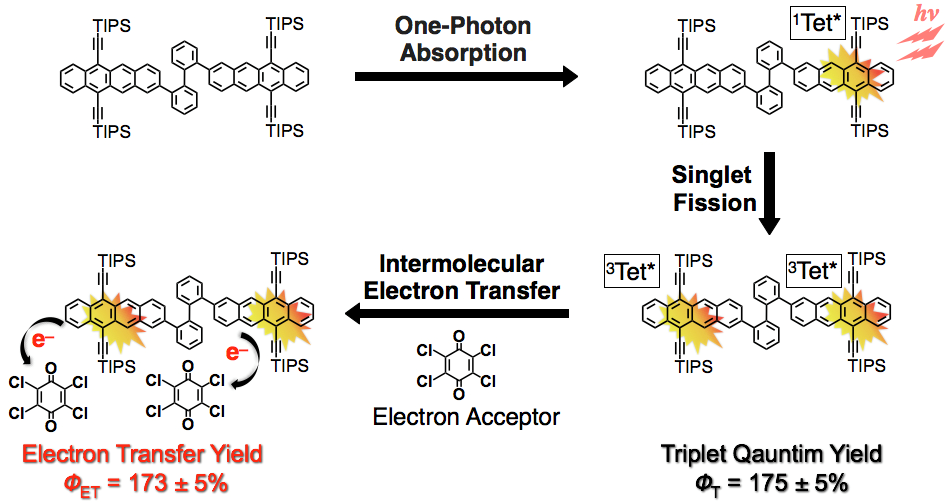
Sequential photoenergy conversion process from singlet fission to intermolecular electron transfer in this study © Keio University
In molecular materials, the absorption of one photon can result in the formation of a singlet exciton ― a state formed by an electron and a hole bound by their electrostatic attraction and with opposite spins. In a process called singlet fission, the singlet exciton splits into two triplet excitons, that is, two excitons in which the electron and hole have their spins pointing in the same direction. The triplet excitons are initially strongly correlated, but they can be separated, and the electrons they carry can then be transferred to other molecules. This complex process is very promising for applications in solar energy conversion, because it opens up the opportunity of reaching very high power conversion efficiencies, as the absorption of a single photon results in the generation of multiple electrical charges (i.e., multi-exciton generation).
Singlet fission can happen within a single molecule in systems of two molecular units covalently linked by an organic bridge (the process in this case is called intramolecular singlet fission). This is advantageous because we can quantitatively evaluate the photophysical processes and parameters such as kinetic constants and quantum yields. Singlet fission theoretically enables the performance of the sequential photoenergy conversion process starting from the singlet state and leading to electron transfer with the radical ion pair quantum yield approaching 200%. However, the quantitative two-electron transfer process through singlet fission has yet to be reported.
Shunta Nakamura, Hayato Sakai, and Taku Hasobe of Keio University, together with colleagues from Kobe University and Tempere University of Technology (Finland) have quantitatively characterized a sequential process involving intramolecular singlet fission and intermolecular two-electron transfer by using two tetracene-based molecules linked by a biphenyl unit (Tet-BP-Tet) as the singlet fission and electron donor system, and chloranil as the electron acceptor system.
The structure of Tet-BP-Tet was studied theoretically by density functional theory, and experimentally by absorption and fluorescence spectroscopy. Femtosecond, nanosecond, and microsecond transient absorption was used to characterize the ultrafast excited-state dynamics and measure the rate of intramolecular singleton fission, the triplet quantum yield, and the rate of dissociation of the triplet-triplet state into individual triplet states. Finally, the long lifetime of the triplet exciton, which is important for the electron transfer to happen, was verified. Performing experiments spanning very different timescales allowed the observation of each of these processes, which happen at very different speeds. Next, the intermolecular electron transfer process was studied, and its rate constant and yield were estimated.
Thus, the authors thoroughly characterized the sequential photoenergy conversion process from intramolecular singlet fission to intermolecular two-electron transfers. As they comment in the paper, "such a molecular system provides a new perspective for construction of future solar energy conversion systems."
Published online 27 March 2019
About the researcher
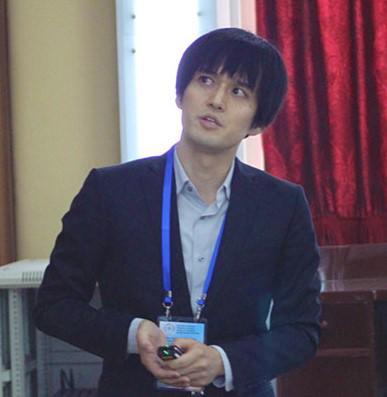
Taku Hasobe― Associate Professor
Department of Chemistry, Faculty of Science and TechnologyAfter receiving his PhD in 2004 from Osaka University, Taku Hasobe worked as a postdoctoral research fellow at the University of Notre Dame, USA. In 2005, he started his academic career at JAIST, and in 2010 he moved to Keio University as an associate professor.
Links
Reference
- Shunta Nakamura, Hayato Sakai, Hiroki Nagashima, Yasuhiro Kobori, Nikolai V. Tkachenko, and Taku Hasobe, Quantitative Sequential Photoenergy Conversion Process from Singlet Fission to Intermolecular Two-Electron Transfers Utilizing Tetracene Dimer. ACS Energy Lett. 4, 26−31 (2019). | article