Scientists in Japan have uncovered novel physics controlling spin-based electronics
Published online 6 March 2015
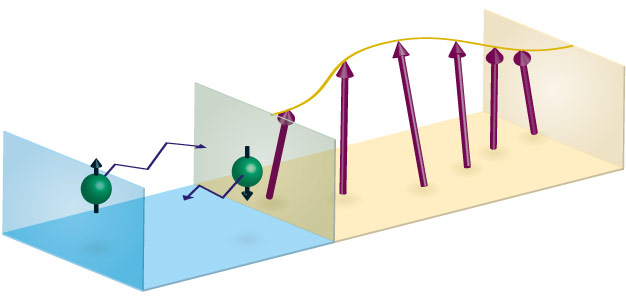
Diffusing electrons in a metal (blue) establish a collective spin excitation in a neighboring insulator (beige) for the long-distance transmission of spin information in spintronic devices.
© 2015 Kazuya Ando, Keio University
As successful as modern electronics has become, it cannot overcome one fatal flaw: pushing electrons down wires dissipates a lot of power. A new generation of spintronic devices can achieve much-improved power efficiencies by encoding information in electron spin, but they also face a steep challenge ― spin information is quickly lost. Now, Hiroto Sakimura, Takaharu Tashiro and Kazuya Ando of the Department of Applied Physics and Physico-Informatics at Keio University in Japan have uncovered physics that may help make the long-distance transmission of spin information a reality1.
The researchers focused on a spintronic system in which an electrical signal in a metal contact induces a traveling spin wave in a neighboring insulating wire (see image). This wave is a collective excitation of the spins of many immobile electrons and comes in discrete chunks (or quasiparticles) called magnons. The wave can travel long distances down the wire before creating a readout current in a second metal contact, which makes the system suitable for transmitting spin information in realistic devices.
Various unconventional techniques exist for controlling the size of the spin current flowing through the insulating wire. Among these is the process of splitting a single magnon into two magnons to increase the spin current. Interactions between magnons have also been observed to increase the magnitude of the current. Researchers, however, have been unclear about the underlying physics driving these enhancements.
Ando and his colleagues used the inverse spin Hall effect, in which a flow of spins creates a constant voltage, to demonstrate that these spin-current enhancements result from an increase in the average magnon lifetime. In the case of a single magnon splitting into two, the increase in steady-state spin current is not, as one might expect, directly the result of the two particles carrying a greater total angular momentum. Instead, magnons interact with each other through a process called spin damping in a way that increases their average lifetime. The researchers found that this process can increase spin currents even in the absence of magnon splitting.
By revealing the central role that magnon lifetimes and magnon-magnon interactions play in determining the magnitude of spin currents, these results may allow for a new way to control signal strengths ― and therefore signal encoding ― in spintronic devices. "The ability to create a spin current is an essential component of any spin-based technology," says Ando. "Our discovery therefore will be the basis for a new generation of spintronic devices."